Untitled Document
Solar System Isotopic Heterogeneity from 53Mn-53Cr
Seann J. McKibbin1, Trevor R. Ireland1 and Yuri Amelin1
1 Research School of Earth Sciences, Australian National University, Canberra, ACT 0200, Australia
Introduction: One of the requirements for the application of the short-lived nuclide chronometer 53Mn (half-life of 3.7 My, anchored to angrite LEW86010) and short lived nuclides in general is that samples are derived from a common reservoir with a homogeneous distribution of the parent nuclide. Ideally, this should reflect the solar system abundances as a whole, but it requires that the solar nebula was well mixed after collapse of the molecular cloud. A more likely scenario is that the solar system is heterogeneous for many isotopes, but particular subsections (such as a specific parent body) will be homogeneous and suitable for short-lived nuclide chronometric studies. Short-lived radionuclide chronometry has been successfully applied and has produced useful information, but many assumptions about initial ratios and ages are involved because insufficient samples have had two or more chronometric systems applied to them. A few samples have been found to have isotope systematics that make them discordant in different systems, showing that a completely uniform distribution of short-lived nuclides in the solar system is not the case [1, 2].
Mn and Cr isotopic heterogeneity: A widespread and systematic chromium isotopic heterogeneity in the inner solar system was recognised by Lugmair and Shukolyukov [1] who found an apparent linear relationship between heliocentric distance and initial 53Cr/52Cr expressed in samples from Earth, Mars and those inferred to originate in the Asteroid Belt. With a few exceptions, meteorites from the Main-Belt have a common 53Cr/52Cr, which suggests that 53Mn/55Mn is homogeneous within the Asteroid Belt and the chronometric interpretation of 53Mn/55Mn for samples from this region is justified. An anomalous case is that of the enstatite chondrites: Shukolyukov and Lugmair [3] showed that they have Mn/Cr ratios that are chondritic, coupled with 53Cr/52Cr ratios that are lower than those of all other meteorites believed to come from the Asteroid Belt. Chondritic Mn/Cr shows that elemental fractionation has not suppressed the evolution of 53Cr/52Cr in enstatite chondrites, so either 53Mn/55Mn or 53Cr/52Cr must be heterogeneous in the solar nebula.
Simple explanations of the radial trend could involve a stellar-derived nucleosynthetic heterogeneity in 53Cr/52Cr, or heterogeneity in 53Mn/55Mn, with either 53Cr or 53Mn increasing with heliocentric distance. Shukolyukov and Lugmair [4] noted that 54Cr anomalies were not observed in meteorites other than carbonaceous chondrites, so anomalies of 53Cr were probably absent as well. Recent 54Cr data does show heterogeneity in the solar system for this isotope [5], but it is not correlated with radial heliocentric distance. Elemental volatility leading to a depletion in Mn (and hence 53Mn) must also be considered. Mn and Cr are approximately in the middle of the volatility range, with Cr being referred to as a common element (50% TC 1296 K) and Mn a moderately volatile element (50% TC 1158 K) [6]. Difference in volatilities is not a favoured explanation because the bulk compositions of the planets are not well known and few constraints can be put on such models.
Comparison with 26Al and Pb-Pb timescales: Though CAIs are used as the reference point for 26Al/27Al, the 53Mn/55Mn ratios obtained from CAIs are inconsistent with the rest of the 53Mn timescale [e.g. 7, 8] (Figure 1) and their generally anomalous isotopic composition suggests that they should not be used as anchors for short-lived nuclide chronometric systems. CAIs have average initial 53Mn/55Mn of 4.4 x 10-5 [9] although values as high as 14.8 x 10-5 have been reported [10]. As CAIs are known to have been isotopically disturbed, these values may represent a lower limit. Using the average 53Mn/55Mn value, this equates to a CAI age that is ~19 Ma older than LEW86010, implying a solar system timescale that is irreconcilably long. The Pb-Pb dates for Efremovka CAIs and LEW86010 [11, 12] give a much smaller age difference of 9.4 ± 1.1 Ma, which is consistent with the 26Al timescale, as well as theoretical models and astronomical observations of stellar system formation. The high initial 53Mn/55Mn may reflect an extreme early heterogeneity in the early solar system [1], which had been largely smoothed over by the time of chondrule formation.
Discrepancies between 26Al and 53Mn ages of some samples (D'Orbigny, Asuka 881394 and others; see [2]) are possibly expressions of solar system heterogeneity for these isotopes, or resolved differences in closure temperature. Placement of samples in the absolute timescale is also problematic, as Pb-Pb dates often have poor precision and different phases commonly return different ages. The most recent efforts at Pb-Pb dating of phases from D'Orbigny [13, 14] have yielded precise ages that are older than previously found, and make this meteorite discordant with the 26Al-timescale. As such, a unique reconciliation of Pb-Pb, Al-Mg, and Mn-Cr isotope systematics is not possible with the data presently available.
Using SHRIMP to obtain 53Mn/55Mn: Sensitive High-mass Resolution Ion Micro-Probe has the potential to obtain initial 53Mn/55Mn ratios for coexisting minerals. This is especially important for chondritic meteorites which are effectively sedimentary rocks or breccias; in these cases bulk-rock samples will not necessarily meet the requirements of internal isochrons and in situ analysis of individual chondrules is preferred. Success will hinge on the precision of 53Cr/52Cr ratios obtained by SHRIMP, and the range of 55Mn/52Cr in coexisting mineral phases (Figure 1).
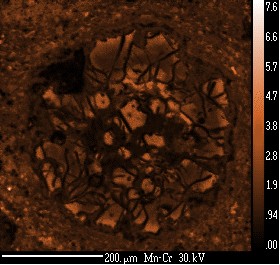
Figure 1. X-ray map of Mn/Cr ratio (calculated from electron probe raw counts) for an unequilibrated olivine chondrule from the Allende carbonaceous chondrite. Coexisting high and low Mn/Cr domains allows determination of 53Mn/55Mn at the time of isotopic closure.
Lugmair G. W. and Shukolyukov A. (1998) Geochimica et Cosmochimica Acta, 62, 2863-2886.
Gounelle M. and Russell S. S. (2005) Geochimica et Cosmochimica Acta, 69, 3129-3144.
Shukolyukov A. and Lugmair G. W. (2004) Geochimica et Cosmochimica Acta, 68, 2875-2888.
Shukolyukov A. and Lugmair G. W. (2000) Space Sci. Rev., 92, 225-236.
Trinquier A., Birck J-L. and Allègre C. J. (2007) Astrophys. J., 655, 1179-1185.
Lodders K. (2003) Astrophysics Journal, 591, 1220-1247.
Lugmair G. W., MacIsaac C. and Shukolyukov A. (1992) LPS XXIII, 823-824.
Nyquist L. E., Bansal B., Wiesmann H. and Shih C-Y. (1994) Meteoritics, 29, 872-885.
Birck J-L. and Allègre C. J. (1988) Nature, 331, 579-584.
Papanastassiou D. A., Wasserburg G. J. and Bogdanovski O. (2005) LPS XXXVI, Abstract #2198.
Amelin Y., Krot A. N., Hutcheon I. D. and Ulyanov A. A. (2002) Science, 297, 1678-1683.
Lugmair G. W. and Galer S. J. G. (1992) Geochimica et Cosmochimica Acta, 56, 1673-1694.
Zartman R. E., Jagoutz E. and Bowring S. A. (2006) LPS XXXVII, Abstract #1580.
Amelin Y. (2007) LPS XXXVIII, Abstract #1669.